Your microbiome is super important to your health, and you can control it by changing your lifestyle, what you eat, how active you are, etc. People writing about the microbiome focus on named organisms, like Akkermansia muciniphila or Escherichia coli, and labeling them as good bugs or bad bugs. In reality, though, the same organism can be good today, bad tomorrow, and be neutral the day after. So, to really make sense of your microbiome and make lifestyle changes, you have to understand what your microbiome is doing (biochemically) rather than just the names of the organisms. Read on to find out more!
Each of us is a walking ecosystem
Please meet your microbiome (micro=tiny, biome=living organisms), a collection of a vast number of different kinds of microbes that live on and inside of you. There is the well-known gut microbiome, but there is also the oral, skin, vaginal, etc. microbiomes. An average human microbiome contains about 50 trillion microbes from more than a thousand species; most of them are bacteria, but we also live with archaea, viruses, fungi, protozoa, and parasites. The human microbiome is like a complex rainforest ecosystem, teeming with diverse life forms, with dynamic interconnected networks of biochemical activity. Just as rainforests are essential for the health of our planet, it is becoming clear to scientists that the microbiome is essential for the health of our bodies.
Most of us have probably been taught that germs are bad and we should destroy them all. However, in the last decade, we have learned that the vast majority of our microbiome is actually beneficial to us – for example, microbes generate vitamin K and some B vitamins, as well as certain bioactive metabolites that are anti-inflammatory. Many researchers have tried to label some species ‘good bugs’ and some other species ‘bad bugs’, but the reality is far more complicated. The same microbe could be a good bug today and potentially a bad bug tomorrow, or just be occupying space and be neutral - 'glad to be here’. So how can we know what exactly these bugs are doing?
Deciphering what microbial genes are actually doing
A gene is a stretch of a DNA sequence that is a blueprint for an RNA molecule of the same sequence. When a gene is activated, or expressed, it is copied into an RNA molecule (also known as a transcript). RNA is then translated into proteins that perform the function encoded by that gene. For example, a particular gene in the E.coli bacterium’s genome could encode for the protein to generate an inflammatory molecule (such as a Lipopolysaccharide, or LPS). But it is important to highlight that just because this gene exists in E.coli does not mean that it is expressed. In fact, most genes are never expressed in a given cell (whether human or microbial) at any time in their life. Just like you have a lot of thoughts in your head, but you speak out only some of those thoughts loudly, and actually act out (express) an even smaller number of your thoughts.
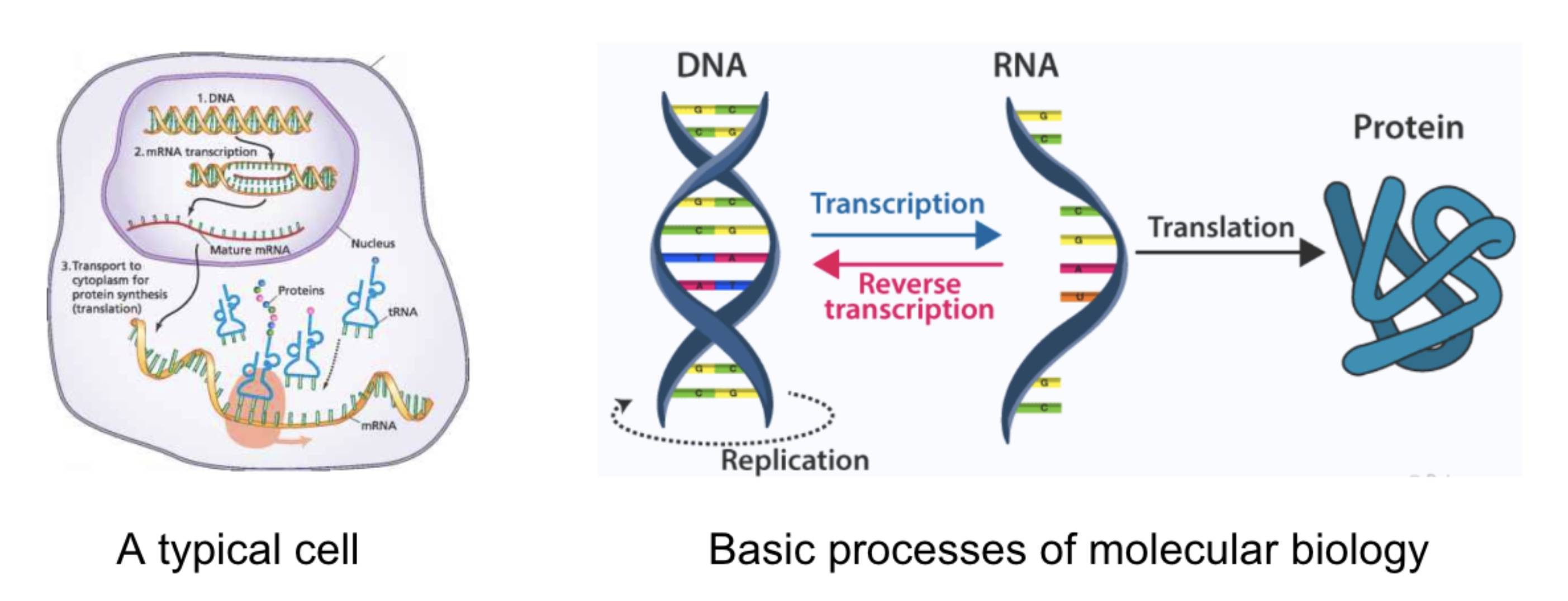
It is estimated that the genomes of the human microbiome collectively contain approximately 46,000,000 genes across the entire human population, with any one person hosting more than 2 million microbial genes (1)(2). However, less than 10% of those microbial genes are actually expressed as RNA to an appreciable level at any given time.
At Viome, we detect and quantify the RNA expression within many sample types (stool, saliva, etc) of microbial genes using a high-resolution metatranscriptomics method (3). In the gut microbiome measured from the stool samples collected from hundreds of thousands of individuals from more than a 100 countries in the current Viome cohort, we detect about 1,100 active microbial species on average, expressing about 80,000 genes (transcripts) on average. This is a lot of biochemical activity!
Interacting microbes are a hotbed of biochemical activity
When thinking about the microbiome, the conventional wisdom (i.e., the vast majority of the scientific literature out there) focuses on naming the microbe from the microbial tree of life, aka the phylogenetic tree, which uses multiple levels such as strains, species, genus, family, etc. For example, E. coli is the name of a species from the genus Escherichia, family Enterobacteriaceae, order Enterobacteriales, class Gammaproteobacteria, and so on. But it turns out that this taxonomic view provides an incomplete picture at best of the role that the microbiome plays in human health and disease. Our core physiological processes, such as the immune system, mitochondrial functions, etc. are all regulated by the microbiome; but of course, none of these human systems care about the taxonomic names we have given the microbes. The true effects of the microbiome are exerted with the chemicals they produce, such as trimethylamine (TMA), butyrate, phenylacetylglutamine (PAG), lipopolysaccharide (LPS), etc. These chemicals are produced by the gut microbes as a byproduct of their own metabolism - when they chemically process the ingredients from the food that we have consumed. These chemicals are known in the scientific jargon as gut microbial (secondary) metabolites, and are some of the major determinants of human health and disease, including longevity.
In one recent paper (4), the findings convincingly demonstrate that microbial functions (as measured by RNA analysis) are far more important than the taxonomic composition (as measured by DNA). The scientists studied the role of gut microbiome in inflammation, specifically related to inflammatory bowel disease (IBD). While they were unable to identify specific microbial taxa (as measured by DNA) related to inflammation, they could find very clear and relevant gene expression (as measured by RNA analysis only) signals. This type of analysis clearly shows that what our microbes are doing is far more important than their taxonomic names. Furthermore, Viome’s RNA method can identify both active microbial organisms and active microbial functions, getting the best of both worlds.
In the drama enacted in the human microbiome, it often matters less who is on the stage. What really matters is the role each character plays in the story. The characters come and go, but the plot continues to unfold with protagonists, antagonists, and mere onlookers, all of whom serve specific functions. It is the actions of these characters, not their identity, that drive the biological narrative forward.
Whodunnit?! Unlocking the microbial mysteries
The genome of a typical bacterium contains about 3000 genes. That means that a typical bacterium can perform about 3000 biochemical functions (some genes are for purely structural purposes, some genes have the same function, and some genes encode multiple functions). For most bacteria, most genes in their genomes are harmless to the human host. Many genes are even beneficial to the human host. Some genes in some microbes may be potentially harmful to the human host.
Take, for example, Akkermansia muciniphila (Am), one of the favorite members of the human gut microbiome. It has been associated with metabolic health in multiple studies, including randomized controlled trials in humans (5). These benefits seem to be derived from a protein Amuc_1100 (6), but also other proteins that produce ornithine lipids (7). At the same time, Am has also been strongly associated with Parkinson’s disease, Alzheimer’s disease, and multiple sclerosis (MS) (7). In addition to the epidemiological studies, molecular mechanisms of the involvement of Am in MS were revealed, adding to the strength of the evidence. When analyzed by DNA sequencing, both of these Am “versions” look identical; that is because they are the same microorganism at the genome level. What the DNA analysis can tell you is only that Am can be both good and bad for you, but it cannot distinguish between the two dramatically different behaviors in different individuals and different circumstances. What can tell them apart? RNA analysis, of course (also known as metatranscriptomics).
Another good example of the difference between the microbiome composition and function is Escherichia coli, also known as E. coli, one of the most famous (or infamous) bacteria. E. coli shows up in the news from time to time, where it is portrayed as a people killer. However, if you look at the scientific literature (which rarely makes the news), you will find that E. coli can be very beneficial for us, producing the very important vitamin K2 (8) and helping us capture iron from our food (9). The matter gets more complicated very quickly, since E. coli has also been associated with the onset and progression of colorectal cancer (10) (11).
So again, when analyzed by DNA methods, it is often impossible to distinguish good behaviors and bad behaviors of E. coli, because they often can perform both beneficial and detrimental functions based on environmental factors. However, by sequencing their RNA, we can distinguish between those two behaviors, since this approach measures the level of gene activation (expression). Like a prism refracting light into a rainbow of brilliance, RNA analysis illuminates the mysteries of microbial activities with precision and detail.
Are the microbiomes of humans from different parts of the world very different?
Not really, it turns out. They may initially look different when only seeing microbial taxa, but when you dig deeper into the microbial functions (biochemical activities), they are actually doing very similar biochemical activities. Here’s some concrete data.
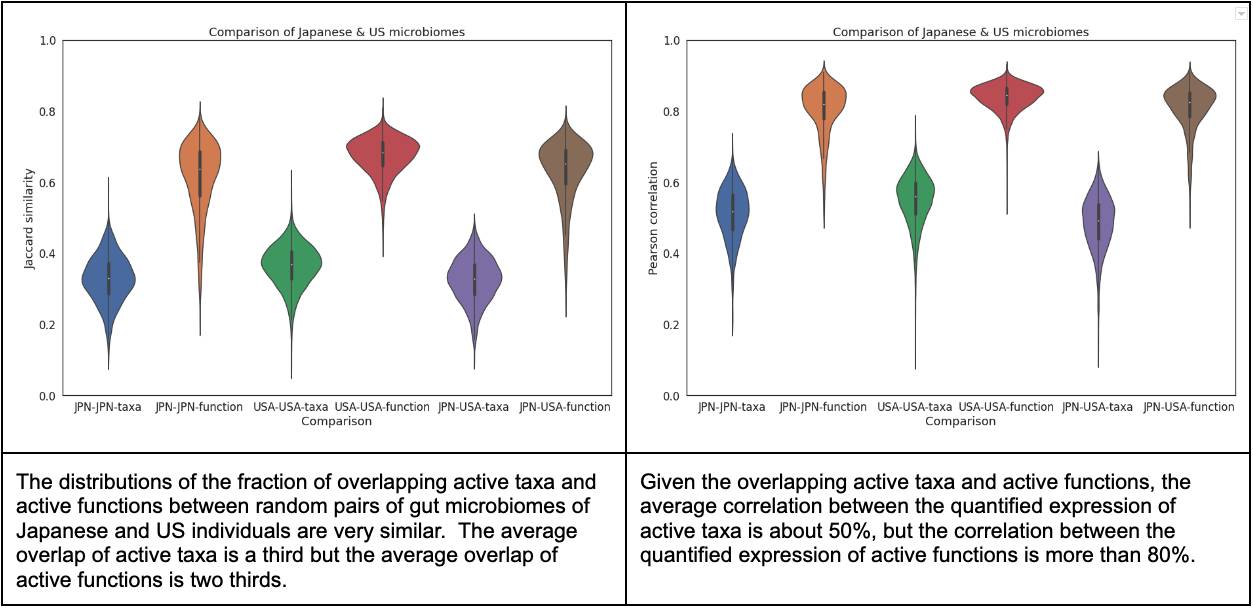
Let’s start by looking at just the presence or absence of activity of taxa and functions in relatively large Japanese (many hundreds) and US populations (many thousands). The chart on the left shows the distributions of the fraction of overlapping active taxa and active functions between random pairs of gut microbiomes of Japanese and US individuals. The average number of overlapping active taxa (species, to be precise) in the gut microbiome of two random Japanese people is 465 out of 1408 (~33%), and the number of overlapping active gene functions (KEGG Orthologs, to be precise) is 2352 out of 3926 (~60%). The average number of overlapping active taxa between two random American people is 533 out of 1454 (~37%), and the number of overlapping active gene functions is 2594 out of 3856 (~67%). And finally, the average number of overlapping active taxa between a random Japanese person and a random American person is 484 out of 1455 (~33%), and the number of overlapping active gene functions is 2455 out of 3900 (~63%). This shows that the overlap of active taxa and functions are very similar between two individuals, whether they are Japanese or US. But more importantly, the percentage of shared active functions (about two thirds) is twice the percentage of shared active taxa (about one third), meaning any random pair of people whether they are from Japan or the US are functionally (biochemically) a lot more similar than they are from a microbial taxonomic perspective.
Now let us consider only the overlapping active taxa and functions and go a step deeper and compute the correlations between the quantified activity of the active taxa and active functions between the Japanese and US populations. The chart on the right shows the result of comparing randomly sampled pairs of individuals and plotting the distribution of correlations. Here again, the average correlation of microbial functional activity in an American’s gut and a Japanese person’s gut is more than 80% (shown by the dark brown distribution in the violin plot), while the average correlation of microbial species activity between the two is only about 50% (shown by the purple distribution). In fact, this turns out to be no different if we were to compare two American (green and red distributions) or two Japanese individuals (blue and light brown). Even when comparing with a range of different metrics (e.g., Pearson correlation, Spearman correlation, Hellinger distance, Bray-Curtis distance), it turns out that microbial functions are substantially more similar between any two individuals, including US & Japanese populations, compared to microbial species. The same story is true with the oral microbiome, measured from saliva samples (data not shown).
But the point is, no matter how you look at it, simply considering microbial taxonomic names overestimates the differences between any two individuals, compared to looking at the microbial biochemical activity. There is strong evidence that the microbiomes of humans from different parts of the world are likely quite similar from a functional perspective. Just like humans share a vast amount of genetic similarity (human genomes of two random people are 99.9% similar), but the small variations can still have profound effects on individual characteristics, such as physical appearance, susceptibility to certain diseases, and personality traits, the microbial biochemical activities share a vast amount of biochemical similarity, but the small differences between individuals can have a profound impact on their lives.
In summary, the microbiome does indeed make a huge difference for your health. But the effect of your microbiome is not determined by the named organisms, like Akkermansia muciniphila or Escherichia coli, but rather with the chemicals they produce, such as trimethylamine (TMA) and butyrate. These chemicals are produced by the gut microbes when they chemically process the ingredients from the food that we have consumed. Out of the approximately 2 million microbial genes in or on an average human, it is estimated that only about 10% are expressed at any given time. Based on the Viome data from large populations in the US and Japan, we see that two randomly selected people from the US or Japan share about two thirds of their biochemical functions in the gut microbiome (vs only about half that percentage of taxa). And when quantified, those shared biochemical functions are more than four fifths correlated (vs only about half among taxa). This goes to show that there is a significant amount of similarity in the gut microbiomes of people from different parts of the world!
According to our data, that still leaves about a third of the biochemical functions to be uniquely present in an average human, and about a fifth of the shared functions being expressed differently. It is important to note that even the small differences in overall functional activity between two people could be significant enough to determine the differences in their health or illness levels. A person with a considerably higher activity of specific biochemical pathways could mean the difference between being healthy versus having a debilitating chronic disease. We will dive into this point in various blogs in the future.
References
1. Gilbert, J. A. et al. Current understanding of the human microbiome. Nat Med 24, 392–400 (2018).
2. Tierney, B. T. et al. The Landscape of Genetic Content in the Gut and Oral Human Microbiome. Cell Host Microbe 26, 283-295.e8 (2019).
3. Hatch, A. et al. A Robust Metatranscriptomic Technology for Population-Scale Studies of Diet, Gut Microbiome, and Human Health. Int J Genomics 2019, 1718741 (2019).
4. Jovel, J. et al. Metagenomics Versus Metatranscriptomics of the Murine Gut Microbiome for Assessing Microbial Metabolism During Inflammation. Front Microbiol 13, 829378 (2022).
5. Depommier, C. et al. Supplementation with Akkermansia muciniphila in overweight and obese human volunteers: a proof-of-concept exploratory study. Nat Med 25, 1096–1103 (2019).
6. Cirstea, M., Radisavljevic, N. & Finlay, B. B. Good Bug, Bad Bug: Breaking through Microbial Stereotypes. Cell Host Microbe 23, 10–13 (2018).
7. Zhang, Q. et al. Genetic mapping of microbial and host traits reveals production of immunomodulatory lipids by Akkermansia muciniphila in the murine gut. Nat Microbiol 8, 424–440 (2023).
8. Bhattacharyya, D. K., Kwon, O. & Meganathan, R. Vitamin K2 (menaquinone) biosynthesis in Escherichia coli: evidence for the presence of an essential histidine residue in o-succinylbenzoyl coenzyme A synthetase. J Bacteriol 179, 6061–6065 (1997).
9. Qi, B. & Han, M. Microbial Siderophore Enterobactin Promotes Mitochondrial Iron Uptake and Development of the Host via Interaction with ATP Synthase. Cell 175, 571-582.e11 (2018).
10. Mathlouthi, N. E. H., Kriaa, A., Keskes, L. A., Rhimi, M. & Gdoura, R. Virulence Factors in Colorectal Cancer Metagenomes and Association of Microbial Siderophores with Advanced Stages. Microorg 10, 2365 (2022).
11. Dalmasso, G., Cougnoux, A., Delmas, J., Darfeuille-Michaud, A. & Bonnet, R. The bacterial genotoxin colibactin promotes colon tumor growth by modifying the tumor microenvironment. Gut Microbes 5, 675–680 (2014).